An Interview With Eliot Coleman: The Original Organic Pioneer
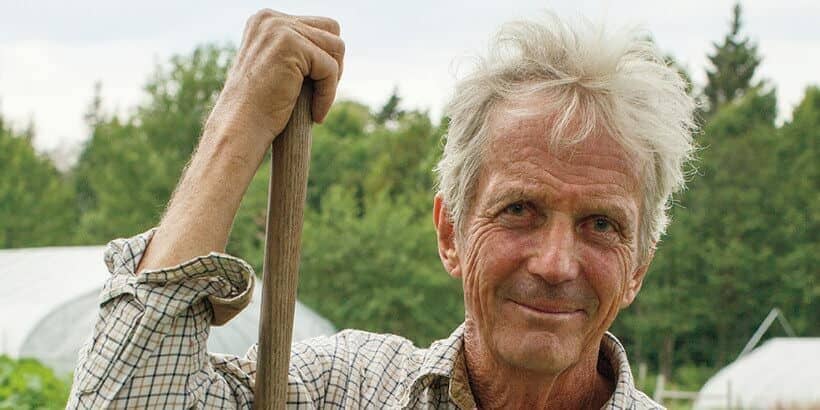
With over fifty years’ experience in agriculture and a penchant for inventing new tools and techniques, Eliot Coleman has solidly established himself as an organic farming pioneer. He has authored several books on his practices, including The Four-Season Harvest, The Winter Harvest Handbook, and his magnum opus, The New Organic Grower, which will celebrate its 30th anniversary this year. Coleman and his wife, Barbara Damrosch, presently operate a commercial year-round market garden at Four Season Farm in Harborside, Maine.
You had been farming for over two decades before the first edition of The New Organic Grower was published. What sparked the decision to record your agricultural experience in the form of a book?
As early as the 1970s, I was teaching informal, weeklong [agricultural] courses, so I’d put together a lot of information on the subject, and I knew where to access the latest studies. Beginning around ’74, I was no longer running my own farm. Instead I was running a farm for someone else and getting paid, which meant that I finally had a little more free time to spend at the word processor. That’s when I began writing The New Organic Grower.
I had always been a book nerd, though. I’ve put together probably the best private library on [organic farming] anywhere in the country. I used to refer to the books on my shelf as my grandparents because they were the ones that taught me how to farm organically—along with the farmers I visited in Europe and the United States seeking more information.
As someone who’s out in the fields working all day, nearly every day, how do you find time to write?
Every evening, every weekend, every bit of spare time. There’s a great book, actually, one of my favorites: The Farming Ladder by George Henderson. Henderson was a farmer in England—one of the best—and he talks about how he was just stealing time away from everything else he was doing to find moments to write.
Did writing The New Organic Grower change the way you farm?
I’d always been maniacal about keeping records, because I thought that was the best way to learn, but I remember saying to myself when I had the first printed copy in my hand, “Damn, I wish I’d had something like this twenty years ago.” What putting it in the form of a book did is coalesce all these disparate ideas into a unit that I could access easily and consult. Prior to that, there’d been God knows how many documents and studies in my file drawers.
What has changed in the world of agriculture in the 30 years since The New Organic Grower was first published?
In ’88 when the first edition came out, I was damn sure that everything I wrote was the right way to farm, and that it made sense. In 2018 I’m even surer than I was then, and even more stunned at the incredible persuasive power of [the agricultural chemical industry], which is making money by preventing people from understanding the benefits of organic farming. That’s what the agricultural chemical companies have done: they try to sell you replacements for what you can get for free.
What advice would you give to young people who are interesting in homesteading, small-scale, and organic farming?
What would I say to young people? All of the angst, all of the ennui in today’s world comes from the fact that peoples’ work—that is, what they do every day for at least eight hours—is unsatisfying. They aren’t aware of that. They don’t know where this itch is coming from. But they know there is an itch that needs to be scratched. The satisfying nature of productive work, where you’re coming out with a product that you can get behind 100%, is of such a nature that there’s just no way that whatever salary you might be earning can equal that. So if you want to look back 50 years from now on a very satisfying life, this is the one you want to look back on.
What are the issues that you find yourself thinking about lately—that you can’t get out of your head?
Right now organic is under assault from big money in food that wants to surreptitiously plant the word organic on the junk they’re producing. Most of the milk and eggs in your supermarket organic section are coming from confined animal feeding operations that do not in any way meet the letter or the sentiment of the law.
The USDA is now also allowing hydroponic produce to be certified as organic without any mention of the fact that it’s hydroponic. So I’m fighting that, because by doing so, the USDA is guilty of grossly defrauding the public—the people who want to purchase organic food. The part that bothers me most is the illegal misrepresentation that denies future generations an accurate understanding of the benefits that organic farming offers to humankind. Organic farming is the only truly sustainable system of agriculture. If you do it right, you don’t need any inputs. The inputs are provided by the boundless energy and logic of the Earth. Organic farming can nourish human beings in perpetuity with exceptional food.
To have that understanding clouded by the fact that the USDA is now allowing the term organic to be put on these totally energy-intensive and non-sustainable products is the thing that bothers me most. As I say in the book, the influence of organic farming over the next ten years—if we follow the paths that I’m suggesting—will blow your socks off as far as understanding our relationship to the natural world, how to preserve it, and how to make sure our species does not totally destroy the Earth as a habitat. Those are the thoughts that go through my head.
Having earned numerous awards and praise from mainstream figures such as Michael Pollan and Martha Stewart, do you feel satisfied with what you’ve accomplished, or is there a drive to continue innovating and pushing boundaries?
The last chapter in the new edition of The New Organic Grower discusses the adventure of organic farming. As an adventurer, you’re always looking for the next journey. So, yes, I’m still trying to design new tools. In fact I have two neat new ideas that I’m presenting at the Common Ground Fair this year. I always tell people that when you’re a climber, the dullest thing is standing on top of the mountain. The fun thing is figuring out how to get there. Farming is figuring out how to get there on a daily basis, which makes it challenging and satisfying.
I take it there’s no retirement plan in the future.
All these people who retire have worked hard all their life at some job they hated, and they finally get old enough to go do what they’d prefer to have done. I’ve been retired since [laughs], since I got into this game, and I’ve been doing what I would prefer to be doing the whole time. So I don’t know what I would retire for. I don’t enjoy sitting on beaches. I don’t take vacations because I find them boring, unless I’m going to visit farms. What makes life stimulating is reality. It’s the doing, and not the sitting around and thinking about it.
Recommended Reads
Recent Articles
Aside from the sheer pleasure of telling your friends, straight-faced, that you maintain your garden using something called a “chicken tractor,” there are a slew of other benefits to working the land with a few of your animal friends. Getting rid of pests without chemicals, for one; letting them do the work of weeding and…
Read MoreIf the idea of running a vegetable farm sounds daunting, you’re not alone. What can you do to simplify techniques and reduce expenses? Where do you even begin?
Read MoreWhen you’re walking around the grocery store looking at the vegetables, it’s probably hard to imagine that a century ago there was twice the amount of options.
Read MoreIf you love tomatoes, you probably already know just how many varieties of these summertime staples there are. But do you know what makes each one unique?
Read MoreAdding the long game of trees to your system results in a deeper and more reliable, resilient and profound presence to your annual vegetable production.
Read More